
Services
GEM has expertise in several research areas of engineering importance.
Multiphysics Simulation
Global Engineering and Materials, Inc. (GEM) has extended its expertise and service areas into (1) physics-based, multi-disciplinary and multi-scale modeling and (2) performance informed processing, operation and maintenance of advanced materials and structures. A suite of problems of different scales ranging from material- to full-scale aircraft structural components have been addressed by GEM’s research and development team. Novel solutions for solving such challenging problems include:
- Development of a process modeling of vacuum assisted resin transfer molding toolkit to optimize composite manufacturing procedures with minimum processing-induced defects while reducing trial-and-error testing on expensive physical prototyping.
- Development of a coupled multi-phase thermodynamics and fluid structure interaction toolkit to optimize the quenching process with coupled thermal, metallurgical, and mechanical properties for reduction of residual stress/distortion in metallic forging parts.
- Development of a multi-physics simulation toolkit for processing tailoring of an additive friction stir deposition to maximize of the fatigue life for repair of corroded metallic structures.
- Development of a novel buffet load response and fatigue damage assessment tool by coupling of an advanced fluid structure interaction module with a hybrid time- and frequency-domain fatigue damage predictor.
Contents
Process Modeling of Vacuum Assisted Resin Transfer Molding
In vacuum assisted resin transfer molding (VARTM), an airbag covers layers of woven fabric of fibers. Air is sucked out from the vent to create a near-vacuum condition inside the airbag. The resin is then injected from the gate to infuse the woven fabric. Once the fabric is fully permeated, high temperature is applied to cure the resin to obtain consolidated part. We use computational fluid dynamics (CFD) simulations to understand the resin infusion process in VARTM. The pressure gradient is established between the gate and vent, which drives the resin flow through the pores within the fabric. The video below shows the infusion for L-shaped beams with different widths. The resin is injected from the upper left corner and is sucked out from the lower right corner.
Modeling Challenge: Dual-Scale Porosity
The fabric consists woven fiber “tows,” which are bundles of individual fibers, as shown below:
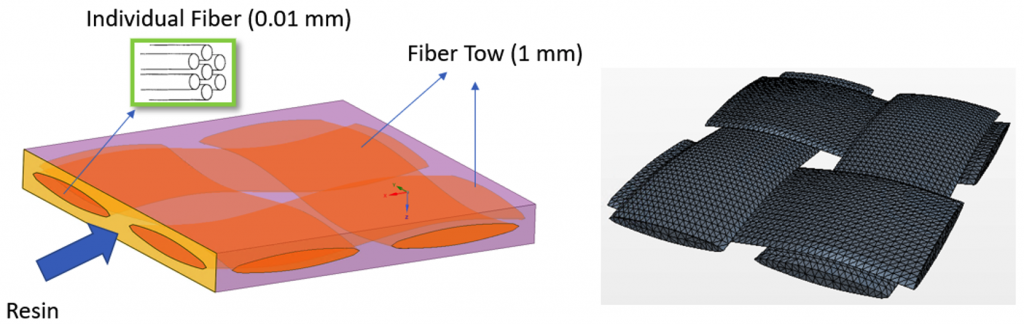
The resin infusion into the tows (intra-tow flow) and around the tows (inter-tow) flow can both be simulated:
When the resin flows in the fabric, it will first flow into the porosity between the tows (inter-tow porosity). After that, the resin can slowly penetrate into the tows through the porosity between individual fibers inside a tow (intra-tow porosity). We introduced a mass sink term in Navier-Stokes equation to capture the absorption of resin into the tows. The videos below shows that the inter-tow pores are first filled, after which it takes a certain amount of time (“bleeding time”) for the intra-tow pores to be filled. The exact form of the sinking term will be obtained by “mesoscale” fluid-structure interaction simulation of the woven fabric (left video above).
Thermal-Metallurgical-Mechanical Modeling of Quenching Process
Under a NAVAIR sponsored program, GEM has been working with University of Illinois Urbana-Champaign and industrial partners to develop a novel multi-physics-based modeling of a quenching process with thermal-metallurgical-mechanical interactions in aluminum components. Because of the complex interaction between temperature, phase-transformation, and stress/strain relation that depends on the temperature distribution and the microstructure of the workpiece, it is essential to implement a performance informed quenching process that can be applied reliably to reduce the high scrap rate of airframe aluminum forging parts with a significant amount of residual stress and distortion. A high-fidelity thermal multi-phase fluid-structure interaction (FSI) model is applied to simulate fluid dynamics and temperature fields in the quenchant tank. The developed immersogeometric modeling approach is used next for an efficient model generation of a 3D workpiece with various dipping orientations. Given the temperature and pressure profiles predicted from the FSI based heat transfer module, residual stress and distortion prediction modules are developed by including temperature and pressure fields mapping and temperature and strain rate dependent property evolution via Abaqus’ user-defined subroutines.
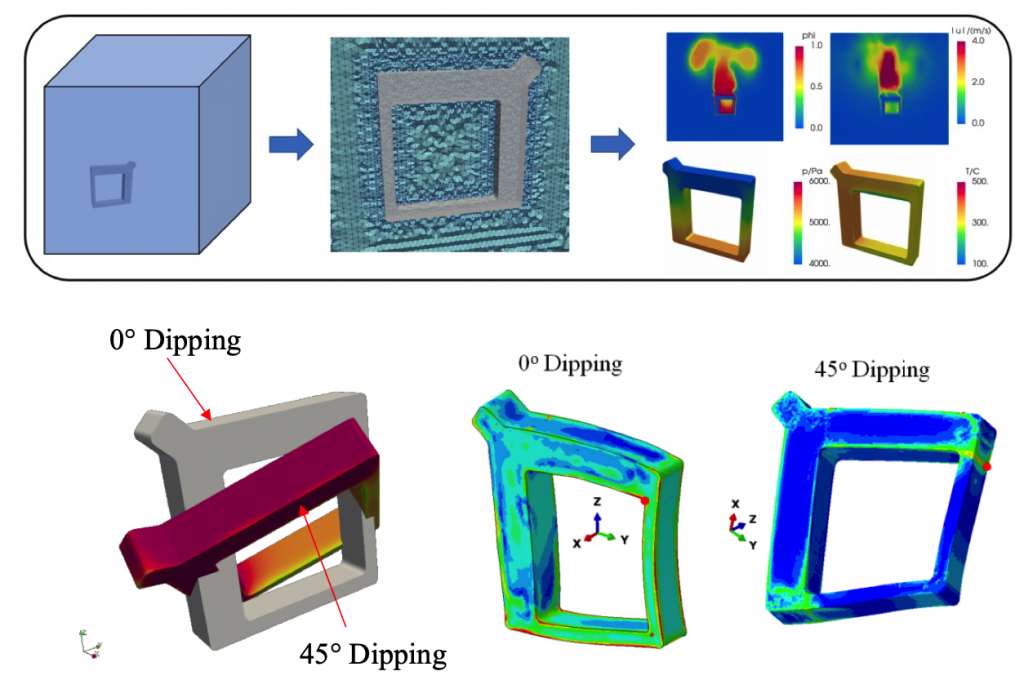
Process-Performance Modeling of Additive Friction Stir Deposition Hole Repair
In Additive Friction Stir Deposition (AFSD) hole repair, we want to repair the corroded surface at the hole in a workpiece. A metal rod is mounted inside a rotating tool, and the rod is fed onto the surface of workpiece while having a high rotational speed. Upon touching the workpiece surface, the metal is plasticly deformed and spread in between the tool shoulder and workpiece. The plastic deformed metal is further squeezed into the hole as the feed rod is continually pushed by an actuator. The hole is re-drilled after the filling, and the corroded surface is repaired via the mixing between the feeding material and workpiece. The material near the repaired hole can have desired mechanical properties due to the refined grains, which is caused by dynamic recrystallization.
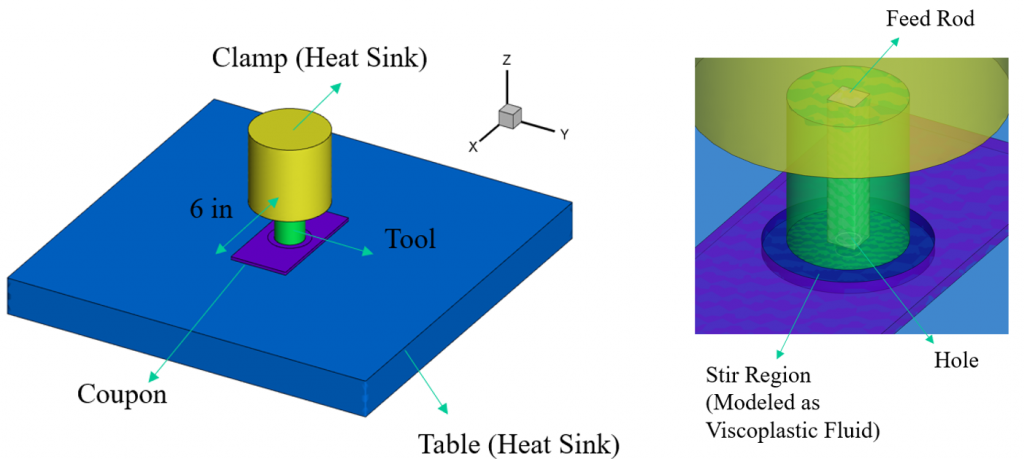
CFD simulation is leveraged to predict temperature and strain rate field (referred to as the thermal-mechanical field) in the plasticly deformed metal, as shown by the figure below. Notice that high temperature and large deformation are resulted from the friction between the tool and workpiece. While the deformation is desired due to its effect to refine grains, the temperature is detrimental as it coarsens the grains and deteriorates the mechanical properties in the deposit.
We developed Abaqus FEM model to predict the fatigue performance based on the property information inferred from the CFD thermal-mechanical field, as shown below. The stress and strain field from a tensile test are predicted, which is used to predict fatigue life. The multiphysics simulation can help understand the relationship among process parameters (e.g., RPM and feed rate), the thermal-mechanical field, grain structure, mechanical properties, and fatigue performance. We can use simulations to minimize trial-and-error tests and guide the selection of process parameters that produce optimal performance.
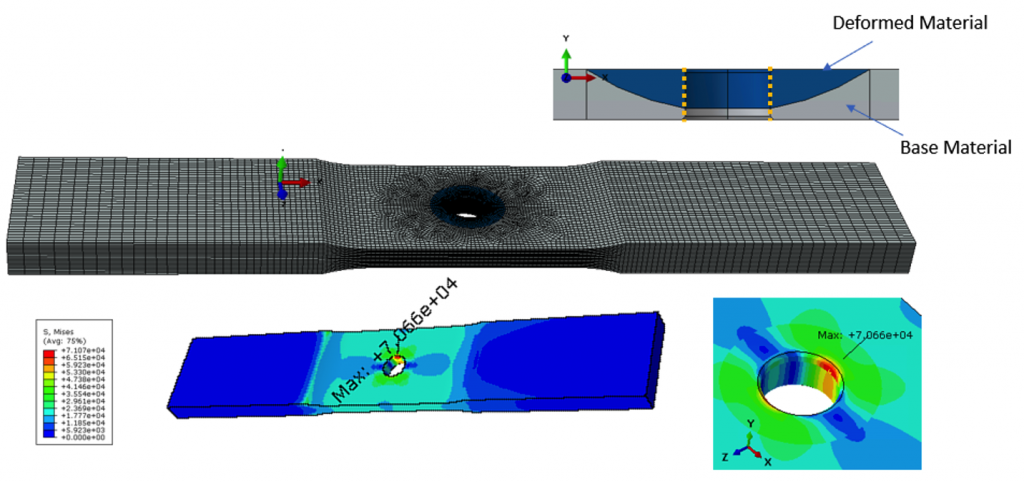
Predictor of Aircraft Structural Load Due to Buffet
Buffet, i.e. large amplitude, low-frequency, and self-sustained shock oscillations of aircraft structures, can result in in-service instability and an expedited accumulation of fatigue damage. Buffet is found to be induced by the interactions between shock-waves and separated shear layers in the transonic regime and can be related to Mach number, angle of attack, aircraft surface geometry, layout of the structural components. etc. Although various experiments and simulations have revealed certain characteristics of the phenomenon, the physical mechanisms are still not fully understood and well predicted. On the other hand, people have been working on the methods based on the in-flight sensor data to predict buffet induced load. One drawback of such kind of approaches is that it is highly dependent on the in-flight data gathered from limited locations. If the sensor data at the critical locations is missing, the prediction can be easily over- or under-estimated. The prediction can also be affected by the poor quality of data collected from sensors due to the presence of noise and a low resolution of the strain gauge signals. Even with a correct given loading profile, the remaining useful life prediction of aircraft structure can still be challenging due to the presence of combined spectrum and spike loading, multi-axial transient loads, and large-scale structures with multiple critical locations.
Under an ongoing NAVAIR sponsored Phase-II program, GEM is currently working to develop a buffet-induced aircraft structural load and multiaxial fatigue damage prediction software (called “BLAST”) via a hybrid immersogeometric (IMGA) and boundary-fitted (BF) compressible-flow fluid-structure interaction (FSI) simulation and the subsequent hybrid time- and frequency-domain multiaxial fatigue damage assessment. An overview of the software capability is provided is the figure below. Starting from the use of IGA based real aircraft geometry for analysis, we only model the critical components (in this case, the horizontal stabilizer) of the aircraft with accurate structural description, while the rest of the structures is treated as fluid boundaries. A hybrid IMGA and BF approach is used to build the fluid mesh such that the entire aircraft is immersed into the background fluid mesh while only the horizontal stabilizer is boundary fitted. As a result, we can add/remove components or modify the aircraft configuration with re-meshing. A maneuvering simulation framework is also developed that allows to simulate the entire flying profile with time-dependent angles of attack. Fully coupled FSI analysis is carried out during the simulation to accurate predict the structural response of the horizontal stabilizer. The recorded stress/strain solutions are then fed into a hybrid fatigue damage analysis module for fatigue damage prediction. The computed data can be used as a reference for maintenance and repair of aircraft structures.
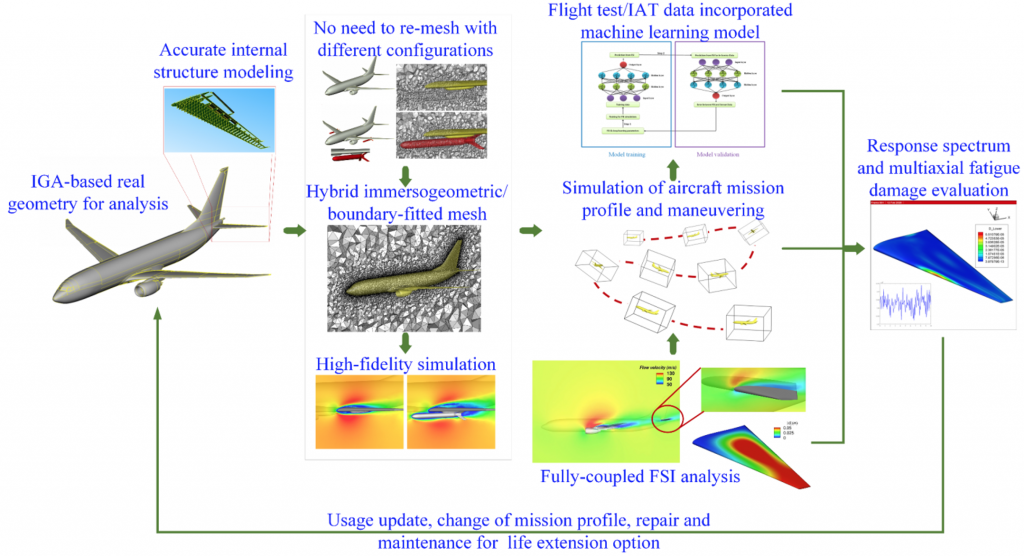